
Fuel Cells
Hydrogen fuel cell stacks
- Home
- Renewable Energy
- Fuel Cells
- Gas Diffusion Layers
Gas Diffusion Layers
Gas diffusion layer (GDL) is an essential component of fuel cells and electrolyzers responsible for a better utilization of the catalyst layer (CL). GDLs are also known as porous transport layer (more general and commonly used in water electrolyzers), liquid/gas diffusion layers (LGDLs), anodic and cathodic distributors, substrates, and electrodes. Gas diffusion layers ensure the uniform distribution of reactive gases on the surface of the electrodes, and they also aid the transport of electrons to or from the external electrical circuit. Gas diffusion layers structurally help the stack withstand the extension caused by the water absorbency. In addition to that, they aid with heat transfer, electrical surface conductivity, and the removal of excess water from the electrodes that is produced by redox reactions. Finally, and most importantly, they clear the path for the fuel and the xxidizing agent towards the catalyst layers.
In summary, their main roles in electrochemical devices include:
- Reactant delivery: Facilitation of efficient and uniform supply of reactants to the catalyst layer, ensuring optimal chemical interactions for the desired reactions.
Product removal: Effective expulsion of reaction by-products from the catalyst layer, promoting a continuous and unimpeded production process.
- Electrical conduction: Seamless transmission of electrical charge across the gas diffusion layer, contributing to enhanced conductivity and improved overall electrochemical performance.
- Heat removal: Efficient dissipation of heat generated during the electrochemical reactions, preventing overheating and maintaining optimal operating temperatures for sustained performance.
- Mechanical support for the catalyst layer: Provision of robust structural support to the catalyst layer, ensuring its integrity and stability during various operational conditions. As an electrode, we combine them with a catalyst ink to create the electrodes that go on either side of the ion exchange membranes.
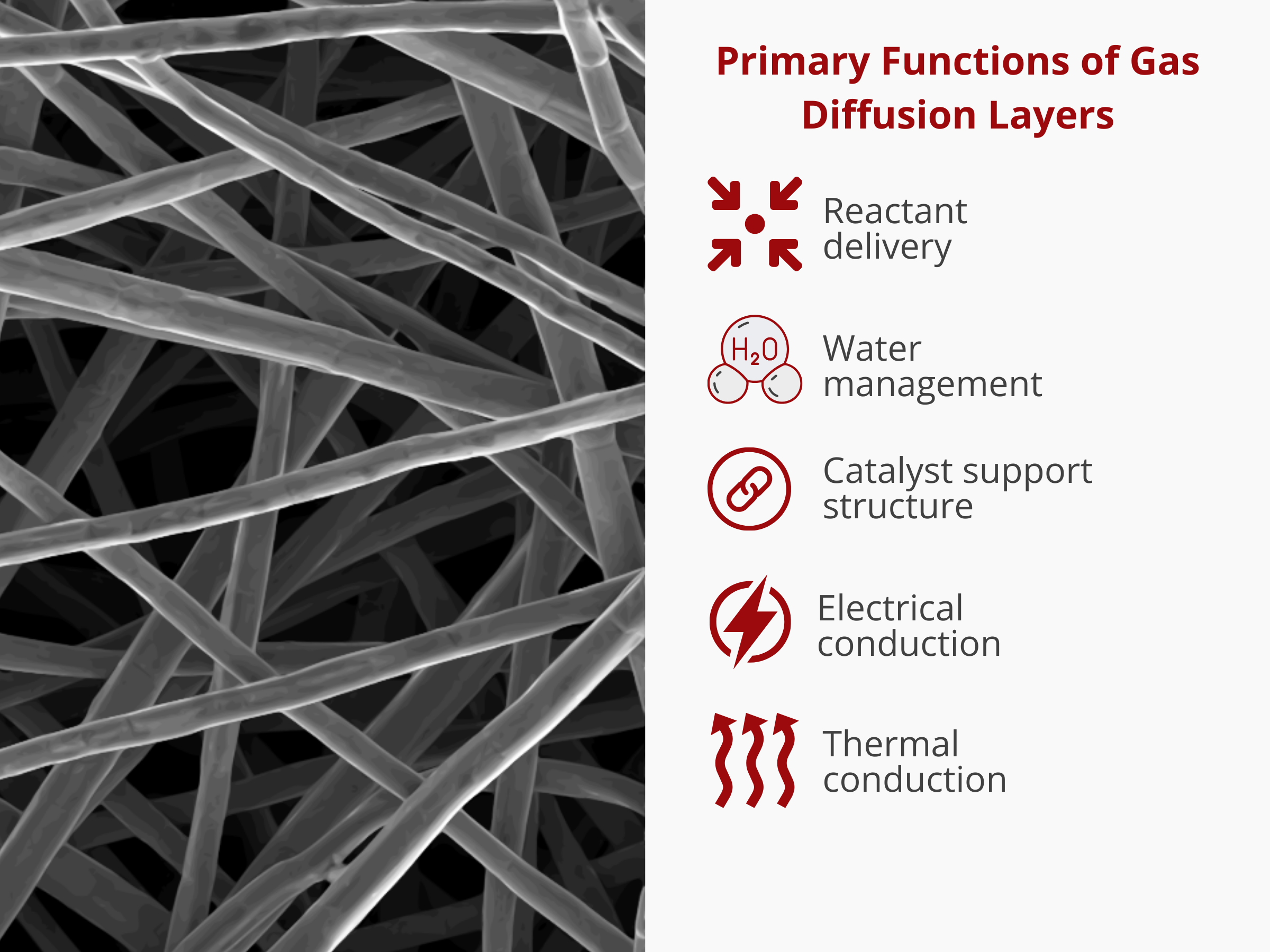
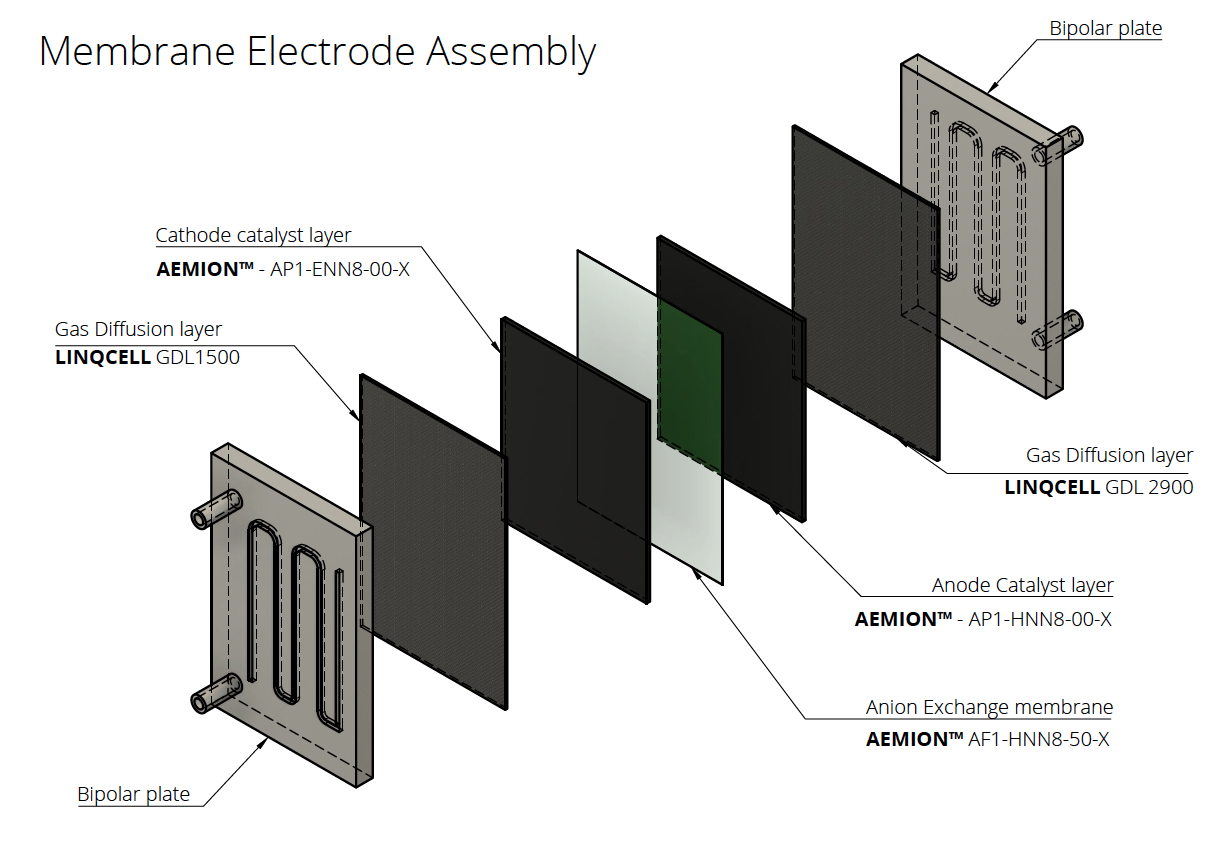
Key Material Property Considerations for Gas Diffusion Layers
Transport Properties of Gas Diffusion Layers
- Gas and Liquid Permeability. Ability of porous media to facilitate the flow of fluid (gas for fuel cells and liquid for water electrolyzers) through its open pores. affected by porosity, pore size distribution, wettability (for liquid permeability)
Electrical Properties. Critical for the efficient functioning of electrochemical devices by providing pathways for electron transfer, maintaining low resistance, and enabling the generation of electrical current during chemical reactions. Often reported as electrical resistance or resistivity or as electrical conductance or conductivity. affected by compressibility, surface roughness, porosity, thickness
- Thermal Conductivity. Measure of how well GDLs conduct heat. affected by porosity, compressive strength, surface treatments, material composition
Structural Properties of Gas Diffusion Layers
Effect of porosity on GDL properties
Porosity, pore distribution and pore volume are important parameters to measure the pore structure of the diffusion layer. Commonly used pore structure measuring instruments are mercury porosimeter and capillary flow porosimeter. The former uses mercury as a wetting fluid, and applies a certain pressure to press the mercury into the hole of the sample to be tested. The latter uses a low surface energy silicone resin (silwick) as the medium, which wets the sample to be tested under the action of capillary force, and then pressurizes it to force it to flow out of the pores.
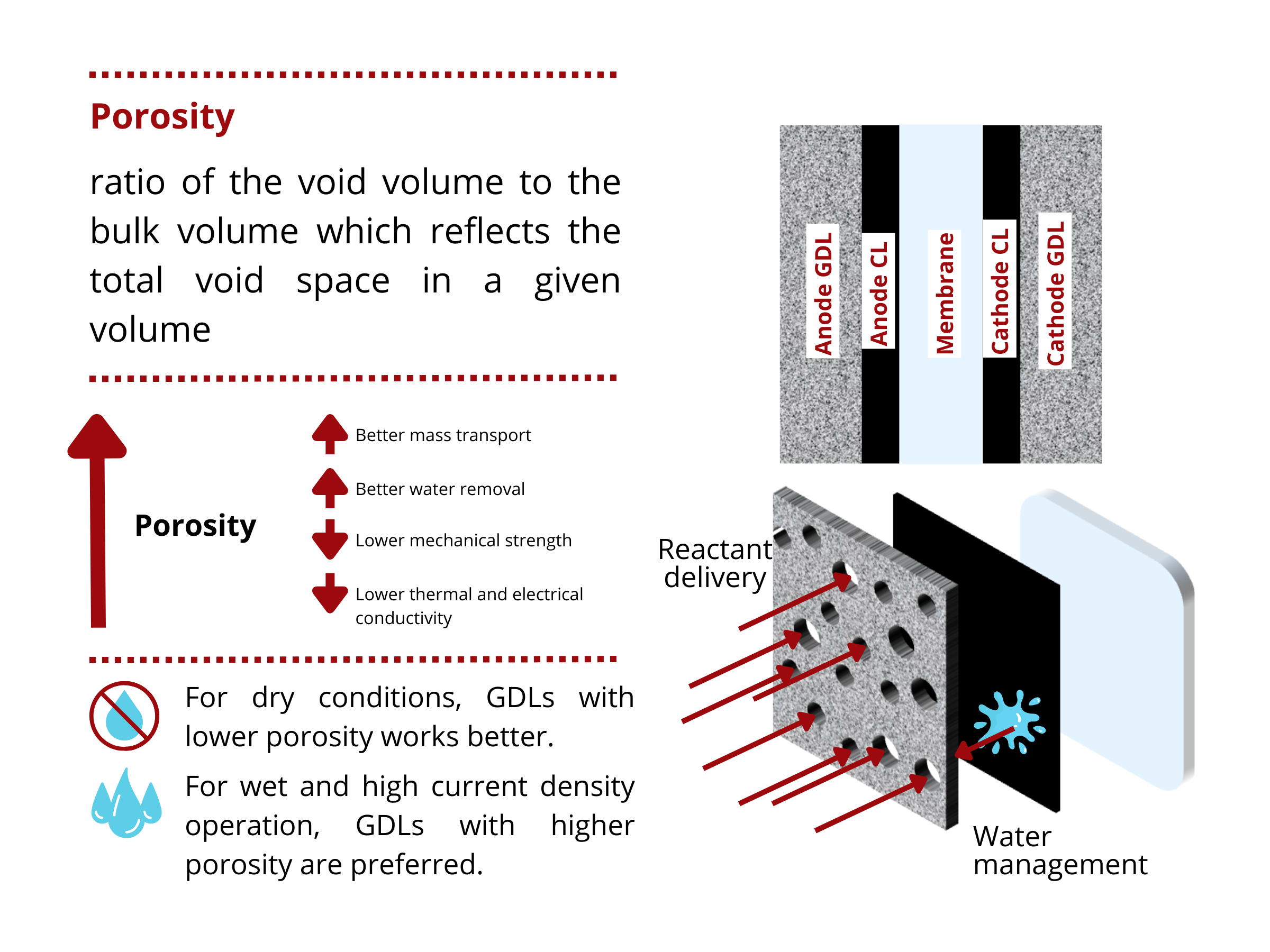
What happens at high GDL porosities?
- High porosities allow the transport of more gaseous reactants to the catalyst layer = HIGH GAS PERMEABILITY
- GDLs with high porosities exhibit high water removal efficiency (or HIGH LIQUID PERMEABILITY), which avoids flooding and the blockage of pores for reactant delivery.
- Higher porosity reduces both the electrical and thermal conductivity of GDLs due to increased void spaces within the material.
- High porosity decreases the mechanical strength of the GDL because there is less solid material for support.
There is no “one size fits all” solution for all water electrolyzer and fuel cell designs. Depending on the cell design and operating conditions, there exists an optimal porosity that balances the mass transport, electrical, thermal, and mechanical properties of the GDL.
How to Balance the Electrical conductivity and Porosity of Carbon-based Gas Diffusion Layers?
Higher porosity in gas diffusion layers (GDLs) decreases their electrical conductivity because the presence of more void spaces within the material means there is less continuous solid material for electrons to travel through.
To counter the effect of high porosity on the electrical performance of GDLs, a microporous layer is sometimes introduced. A microporous layer is a thin, porous material, often composed of carbon or other conductive materials, that is applied to the surface of a gas diffusion layer (GDL) in electrochemical devices. It consists of very small pores, typically on the nanometer scale, and serves to optimize the interface between the GDL and the adjacent catalyst layer.
Effect of thickness on GDL properties
- Thicker GDLs have longer path lengths for water diffusion, which increases the resistance to water penetration.
- Thicker GDLs often have high surface areas within its porous structure, allowing water to be absorbed at more sites.
- the through-plane transport of reactants and water decreases, whereas the in-plane mass transport increases.
- Thicker GDLs have higher through-plane electrical resistance.
Gas Diffusion Layers (GDLs) in Fuel Cells
Gas Diffusion Layers (GDLs) are key components in various types of fuel cells, including:
- Proton Exchange Membrane(PEM) stacks
- Direct Methanol (DMFC) stacks
- Phosphoric Acid (PAFC) stacks
- Electrolyzers
- Redox Flow Batteries
- Other electrochemical devices
In fuel cells, this thin, porous sheet must provide high electrical and thermal conductivity and chemical/corrosion resistance, in addition to controlling the proper flow of reactant gases (hydrogen and air) and managing the water transport out of the membrane electrode assembly (MEA).
This layer must also have controlled compressibility to support the external forces from the assembly, and not deform into the bi-component plate channels to restrict flow.
Other uses require different criteria, for example, electrolyzers require thicker, higher density porous plates, while humidifiers have most of the same requirements as fuel cell stacks, but the GDLs do not need to be electrically conductive.
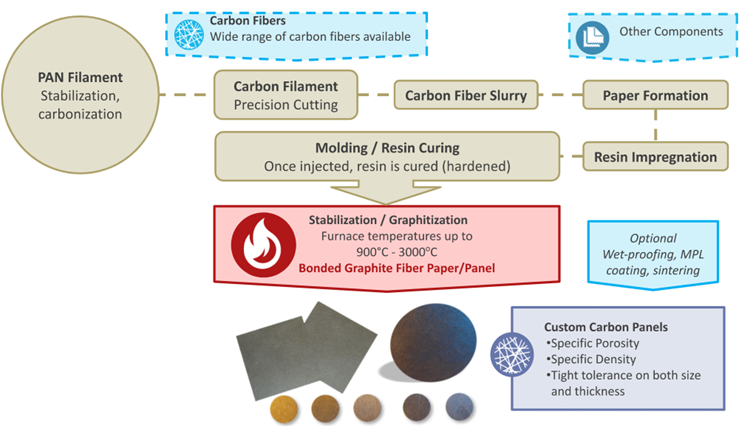
Carbon-based Gas Diffusion Layers
At the cathode side, carbon-based materials are the predominantly used gas diffusion or porous transport layer owing to the stability of carbon under reducing conditions.
Activated Carbon Fabric as Gas Diffusion Layers
Activated Carbon Fabrics (ACF) are high surface area, drapeable cloths that are used in various electrochemical devices including ultracapacitors, electrical double layer capacitors, and battery electrodes. They also serve as the high surface area electrode in many electrochemical purification and electrosorption processes, as well as unique applications such as a carrier for thin film oxides in various devices. They are used in numerous research programs and articles related to energy storage, purification and electrochemical processes.
Our previous popular 2225A Spectracarb grade has been discontinued so we are looking into developing a direct alternative. Contact us for more information.
Sheets, Papers, Plates or Panels as Gas Diffusion Layers
Gas Diffusion Layers are “graphitized“ carbon fiber-based nonwovens known as either carbon sheets, papers, plates or panels. Though the difference in these terms is often trivial, thinner substrates are often called sheets or papers, whereas thicker ones are more often referred to as panels or plates. Both can be machined into "disks" which is often the preferred shape for circular stack-type applications. In all cases, these carbon fiber substrates are specifically designed for use in PEM, DMFC and PAFC fuel cells to transport reactant gases into and excess liquid product water out of the electrocatalyst layers. Their porosity is typically above 70%, leaving enough room for the reactions to take place. Fuel cell GDLs are typically sheets thinner than 1 mm. CAPLINQ can customize thicknesses, but these are the most frequently requested by our customers.
LINQCELL Gas Diffusion Layer (GDL) sheets are optimized for:
Electrical Conductivity
Mechanical Strength and Durability
Gas/Air Permeability
Water Management
Product | Thickness | Thickness (mm) | Density (g/cm3) | Basis weight (g/m2) | Through-Plane Resistance (mΩcm2) | Through-Plane Resistivity (mΩcm) | Voltage Loss (mV) |
---|---|---|---|---|---|---|---|
GDS 180 | 0.007" | 0.18 | - | 50 | 7 | - | - |
GDP 210 | 0.008" | 0.21 | - | 51 | 10 | - | 14 |
GDL 240 | 0.009" | 0.24 | - | 90 | 15 | - | - |
GDP 340 | 0.013" | 0.34 | - | 90 | 10 | - | - |
All values are indicative and subject to tolerance
Click to learn more about GDL Graphitized Carbon Paper & Panels.
For fuel cell configuration and operationg conditions wherein carbon corrosion is expected, metal-based GDLs are used as alternatives. Titanium-based and nickel-based GDLs have been used as the cathode diffusion medium in PEM fuel cells. They have been also utilized as liquid fuel diffusion medium in direct methanol fuel cells and bifunctional oxygen electrode for unitized regenerative fuel cell. The following tables outline the properties of titanium and nickel fiber papers—promising alternatives to traditional carbon paper in fuel cell applications.
LINQCELLTM Titanium Fiber Paper Properties
Product | Porosity (%) | Thickness (um)* | Basis Weight (g) | Actual weight (g)** |
50–60 | 250 | 45.5 | 18.3–22.6 | |
60–70 | 250 | 45.5 | 13.7–18.1 | |
50–60 | 400 | 72.8 | 29–36.3 | |
60–70 | 400 | 72.8 | 22–29 | |
50–60 | 600 | 109.3 | 44–54.5 | |
60–70 | 600 | 109.3 | 32.8–43.6 | |
50–60 | 800 | 145.7 | 58.3–72.9 | |
60–70 | 800 | 145.7 | 43.7–58.2 |
LINQCELLTM Nickel Fiber Paper Properties
Product | Porosity (%) | Thickness (um) | Elongation (%) | Tensile Strength (MPa) |
40–90 | 250 | ≥8 | 5–8 | |
50–90 | 500 | ≥8 | 5–8 |