Batteries
Renewable energy sources have been increasingly integrated into the global energy mix as the initial investment costs for these sources decrease. The shift towards renewable energy aligns with the initiatives in place to replace fossil fuels due to the depleting reserves and the harmful health and environmental effects associated with their continued use. However, the highly variable and intermittent nature of renewable energy sources result in the mismatch between the energy supply and demand. To address this mismatch and maximize the renewable energy capacity, energy storage systems have been integrated to renewable energy sources.1
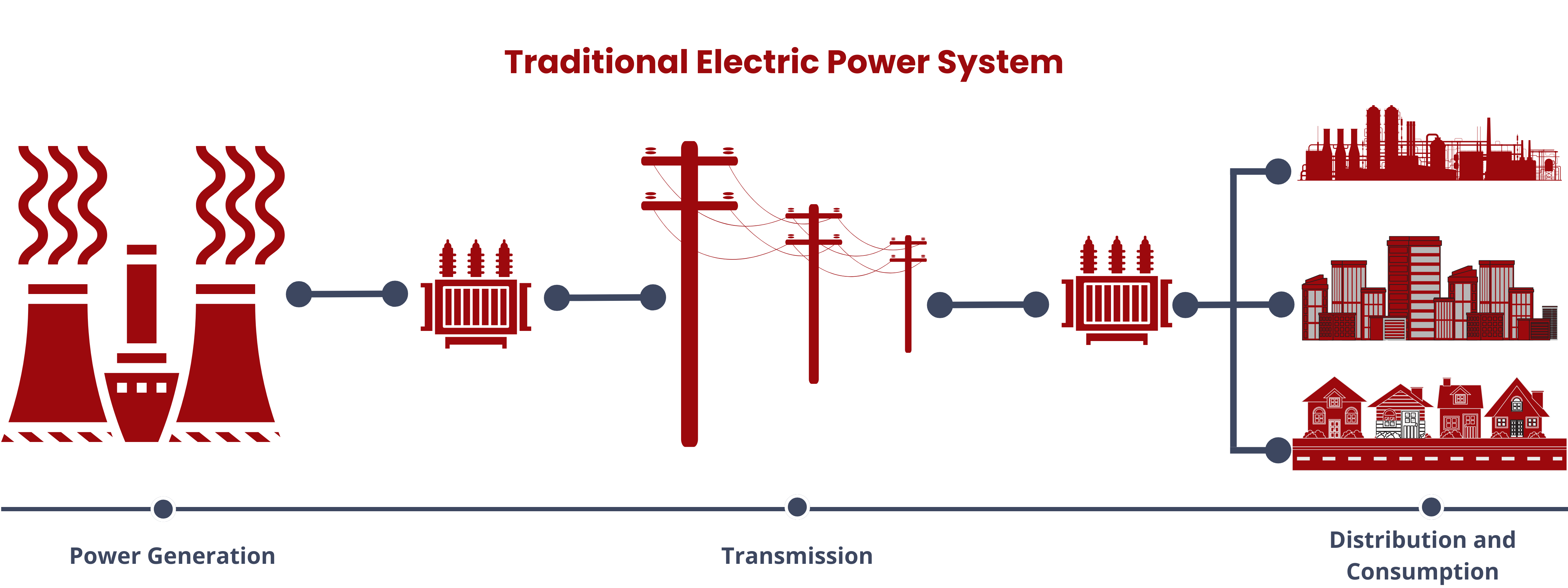
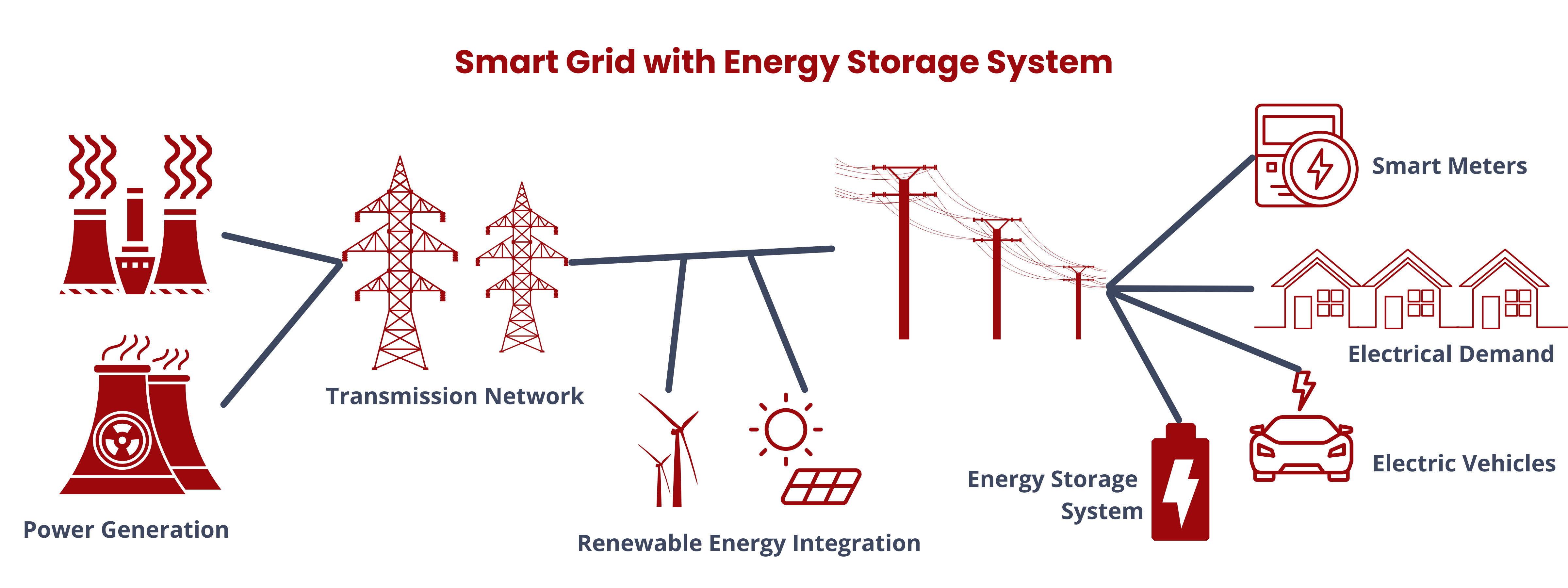
Energy storage systems (ESS) are versatile solutions in modern energy management. They store energy produced at one time for later use, facilitating a bidirectional flow of energy between the device and the power grid during both charging and discharging phases. Aside from allowing the seamless integration of renewable energy sources, ESS also performs the following functions.
- Balances grid supply and demand on various time scales, from seconds to hours, to maintain grid stability and power quality.
- Provides fast response (ramping) to offer ancillary services for grid operators, helping regulate electric grid frequency in real-time.
- Enables peak electricity demand reduction and price arbitrage by storing energy during low-demand periods and discharging it during high-demand times.
- Defers expensive electricity infrastructure investments by managing growing demand more cost-effectively.
- Offers backup power during grid outages for on-grid electricity consumers.
- Reduces end-user electricity demand and associated demand charges, particularly in commercial and industrial settings.
- Integrated with microgrids to enhance reliability and resilience in localized power supply applications.2
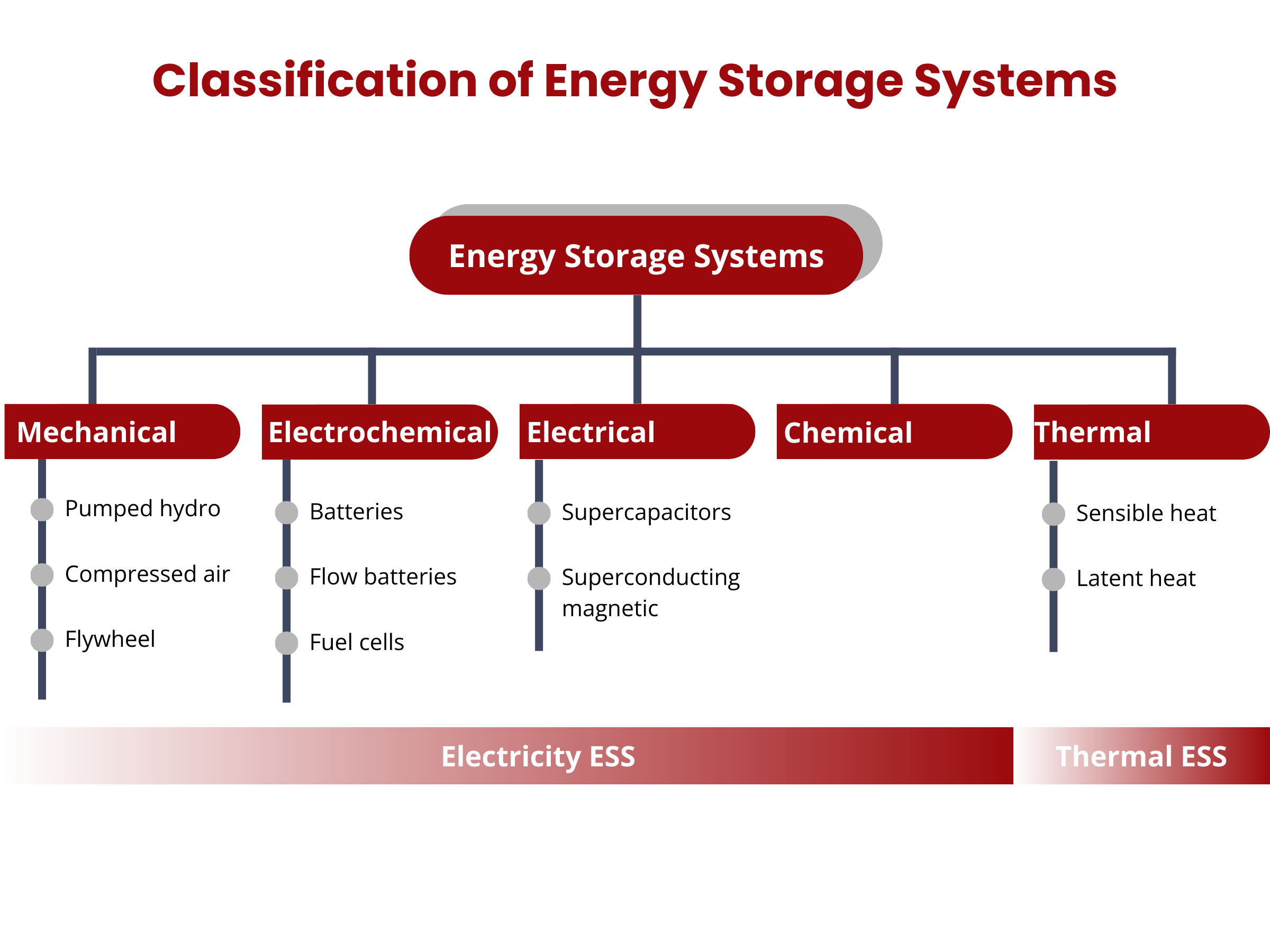
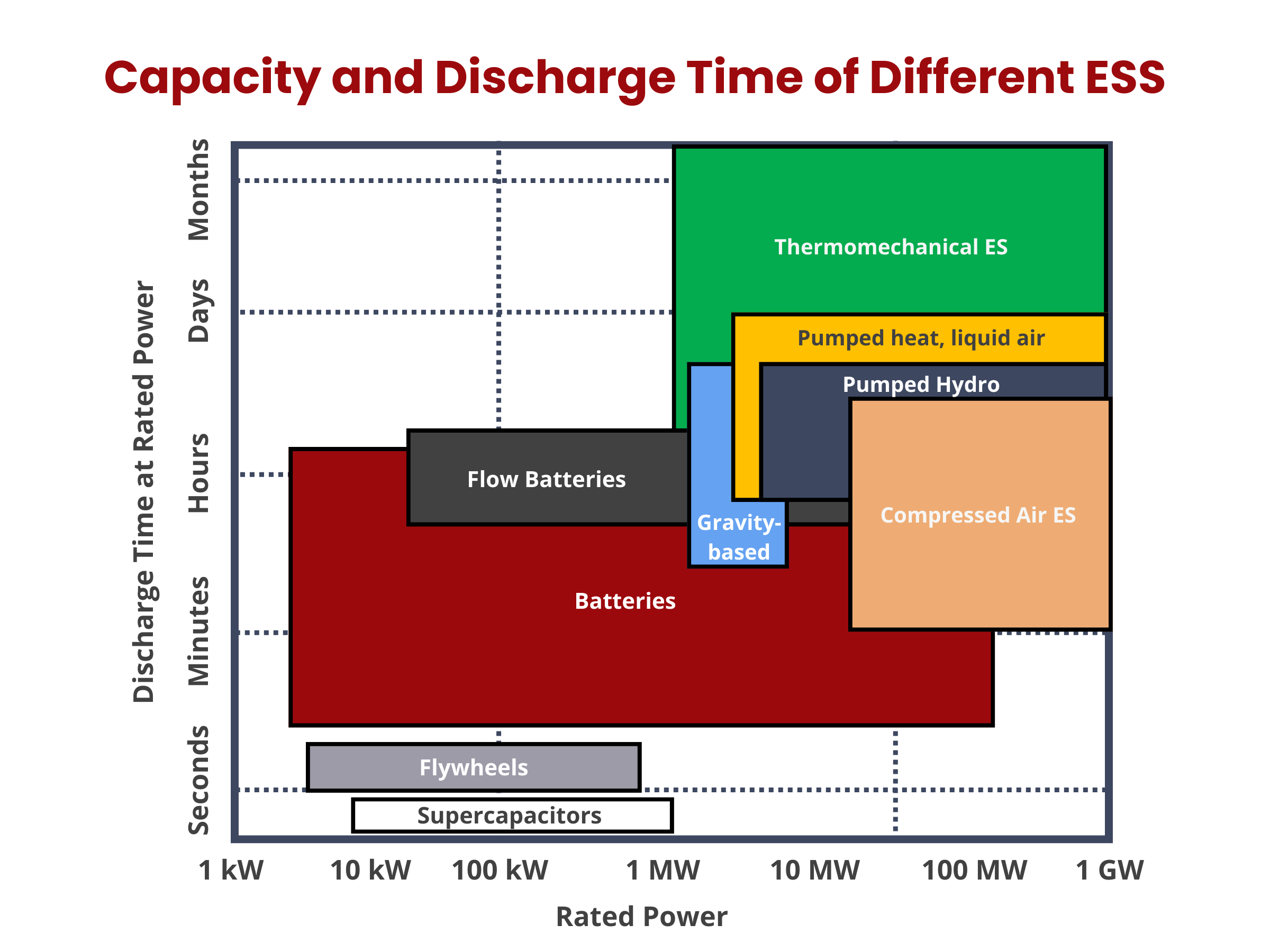
Energy storage systems (ESS) can be broadly categorized into two main groups, based on their integration with power or thermal energy grids: electricity ESS and thermal ESS. Electricity ESS can be further classified into four primary types: electrochemical, electromagnetic, mechanical, and chemical energy storage. Meanwhile, thermal ESS focuses on storing heat or cooling energy in specialized storage tanks.3
Electrochemical energy storage devices are the most traditional EES. Electrochemical EES devices convert stored chemical energy into electrical energy. Unlike that of traditional thermodynamic processes in thermal EES, the efficiency of electrochemical processes is not Carnot-limited. Thermal EES rely on the heat transfer between reservoirs and are constrained by the Carnot limit, whereas electrochemical processes involve the movement of electrons and ions through a chemical medium. This different mechanism allows electrochemical cells to achieve high efficiency without being restricted by the Carnot framework.4
Battery energy storage systems (BESS) as the dominant electrochemical energy storage technology
Electrochemical ESS include primary (non-rechargeable) and secondary (rechargeable) batteries and fuel cells. Among the electrochemical energy storage devices, batteries continue to have a dominant position in the energy market due to their high efficiency and excellent reliability. Batteries can be seamlessly integrated into different applications while requiring minimal maintenance, which is well reflected in global statistics where batteries account for 40% of total global energy output. Projections even indicate a five-fold increase in the consumption over the next decade.1 This growth is driven by their pivotal role in enabling the transition to renewable energy sources and the significant environmental benefits they offer, a trend supported by ongoing research and development efforts.
Batteries, as a form of electrochemical energy storage, are composed of one or more rechargeable cells and have played a transformative role in the transportation and energy sectors. They contribute significantly to reducing greenhouse gas emissions by optimizing production processes, responsibly sourcing raw materials, and advancing recycling and reusing practices for battery components. In microgrid energy storage applications, a variety of battery types are widely utilized, with capacities ranging from several kilowatts to thousands of kilowatts, reflecting their versatility and adaptability to diverse energy storage needs.
Batteries vs. fuel cells
As both are electrochemical devices in nature, the working principles of batteries and fuel cells are similar. The key difference is that fuel cells are open systems that convert chemically bound energy supplied from external fuels, such as hydrogen, methanol, and hydrazine, whereas batteries are closed systems that convert chemical energy integrated into their structure. Not considering material degradation, fuel cells will continue operating as long as fuels are being supplied. Batteries, on the other hand, must be recharged once the stored chemical energy within it has been converted, else, its useful life ends. This fundamental distinction in their energy sources influences their respective roles and applications in various energy and power generation systems.
Aspect | Batteries | Fuel Cells |
---|---|---|
Energy Source | Internal chemical components | External synthetic fuels (e.g., hydrogen, methanol) |
Rechargeability | Yes (rechargeable) | Continuous supply of fuel is needed |
Efficiency | High | High |
Environmental Impact | Can be environmentally friendly | Emissions depend on fuel source |
Energy Density | Lower energy density | Higher energy density |
Application Range | Portable devices, electric vehicles, grid energy storage | Stationary and mobile power generation, transportation (hydrogen fuel cells) |
Storage Capacity | Limited by the size of the battery | Scalable based on fuel supply |
Operating Temperature Range | Wide range | Dependent on the type of fuel cell |
Life Cycle | Limited by charge/discharge cycles | Longer lifespan but fuel-dependent |
Rapid Charging Capability | Varies by battery type | Faster refueling with some fuel cell types |
Size and Weight | Bulky and heavy for high capacity | Can be more compact and lightweight for the same energy output |
Similar to fuel cells, batteries are composed of two electrodes and an electrolyte enclosed within a dedicated container and connected to an external source or load. The electrodes sandwich the electrolyte. This common structure is important because it shows that both batteries and fuel cells follow similar principles in how they work. Even though they operate differently, understanding this shared foundation helps us grasp their roles as devices for storing and converting energy.
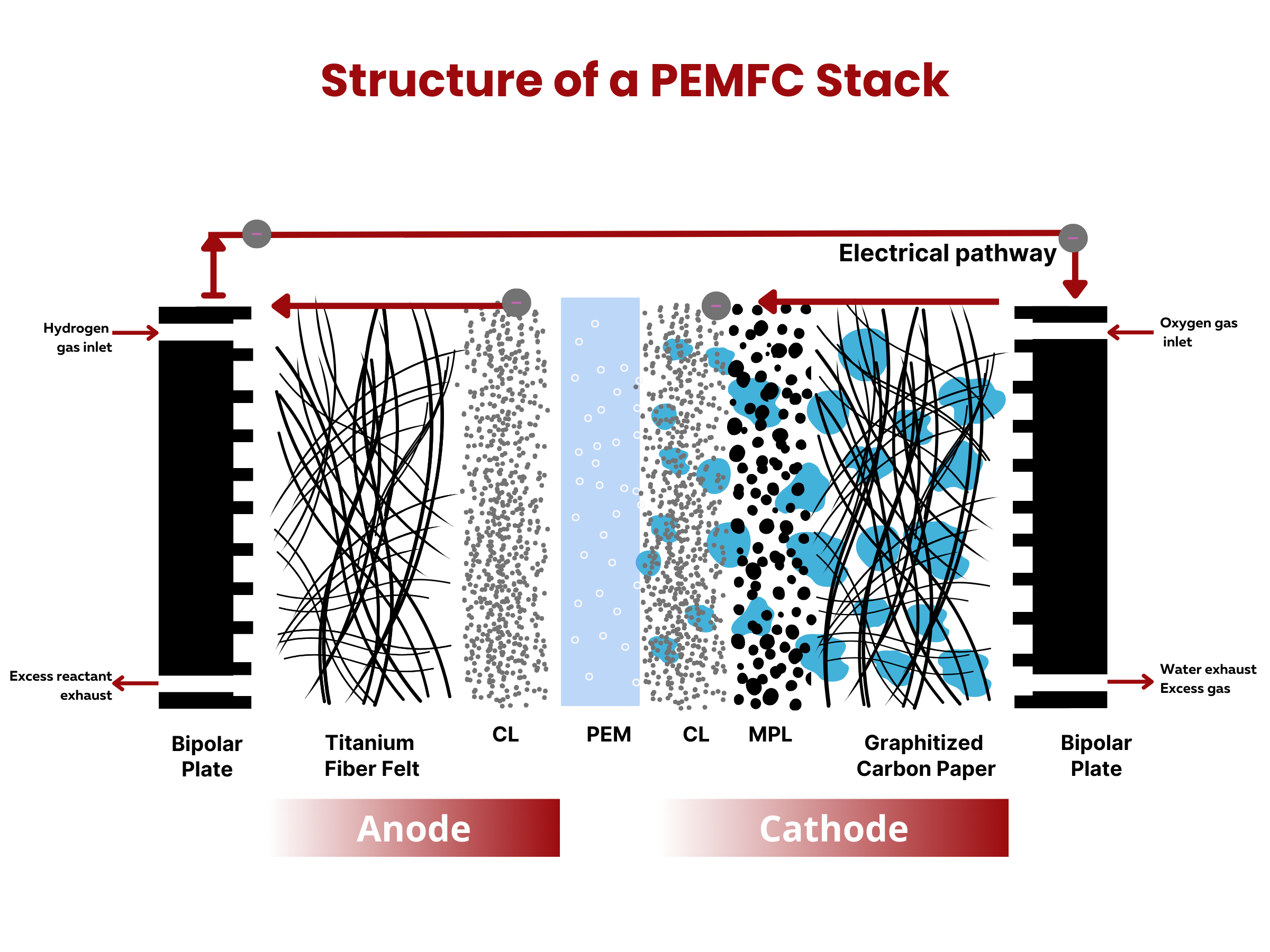
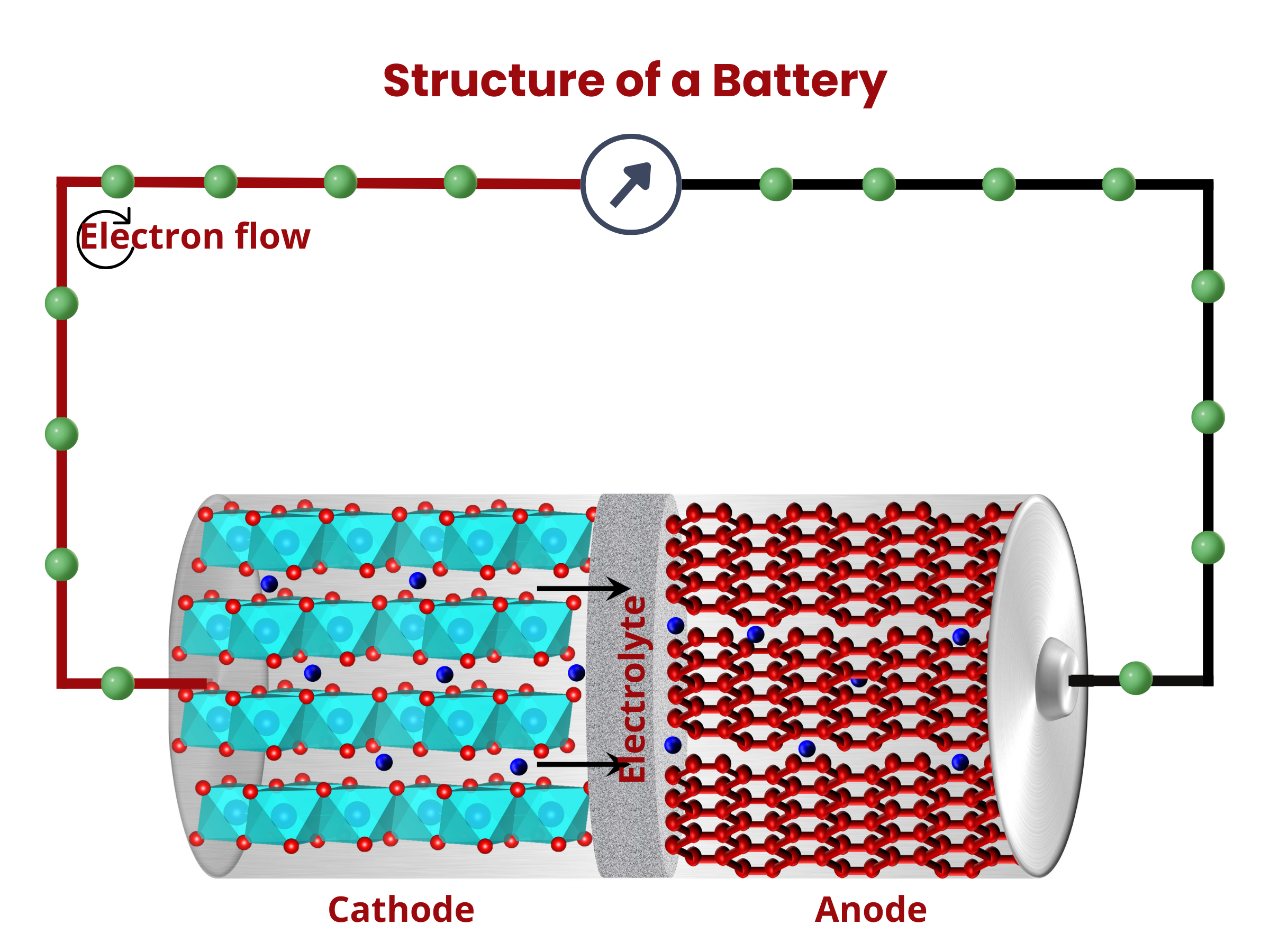
What are the two primary battery classifications?
Batteries can be broadly classified into two categories– primary and secondary batteries. The key distinction lies in the rechargeability of secondary batteries, as opposed to primary batteries, which cannot be recharged. The reactions in primary batteries cannot be easily reversed. As such, when the battery electrodes are used up, they cannot be reverted back to their original state even when an external voltage is applied. On the contrary, secondary batteries can be recharged and used again by applying external voltage. Typically, primary batteries have higher specific energy (in W⋅h⋅kg–1) and power (in W⋅kg–1) than secondary batteries. Side note: specific energy is the energy capacity of the battery per unit battery weight, whereas specific power is the highest power that the battery can produce in a short period of time per unit battery weight.
Feature | Primary Batteries | Secondary Batteries |
Rechargeability | Not rechargeable | Rechargeable |
Typical Chemistry | Alkaline, Zinc–Carbon, Lithium | Nickel–Cadmium (NiCd), Nickel–Metal Hydride (NiMH), Lithium–Ion (Li-ion) |
Usage | Single-use, disposable | Reusable, can be recharged multiple times |
Initial Cost | Generally lower | Higher initial cost, offset by reuse |
Energy Density | Lower energy density compared to some secondary batteries | Higher energy density in some cases |
Environmental Impact | Can contribute to e-waste | Better environmental profile if properly recycled |
Common Applications | Remote controls, flashlights, toys, certain medical devices | Laptops, smartphones, electric vehicles, cameras, power tools, cordless phones, portable audio devices, e-cigarettes, medical devices, uninterruptible power supplies (UPS), renewable energy storage |
How do batteries work?
Inside the battery, chemical reactions, namely oxidation and reduction, occur at the electrodes. During discharging, oxidation occurs at the anode, releasing electrons that travel through the external circuitry to the cathode. At the cathode, these electrons are used up during the reduction reaction. At the same time, ions move through the electrolyte. This movement maintains charge neutrality within the battery. The flow of electron through the external circuit creates an electric current, therefore discharging the battery.
Primary batteries can only be discharged. Once their chemical reactions are depleted or reach a point where they cannot sustain the necessary voltage, the battery is considered "dead" and cannot be recharged. The electrochemical reactions that generate electrical energy in primary batteries are typically not reversible, distinguishing them from secondary batteries, which are rechargeable and allow for repeated charging and discharging cycles.
To charge secondary batteries, an external voltage is applied. This applied voltage reverses the electrochemical processes. The electrons are forced to move from the cathode to the anode, essentially restoring the reactants and allowing the battery to be reused. As long as there are reactants in the anode and cathode, and the circuit is closed, secondary batteries continue to produce electrical energy through the electrochemical reactions. Over time, the chemical reactions deplete the reactants in the anode and cathode, which reduces the battery's ability to generate electrical energy.
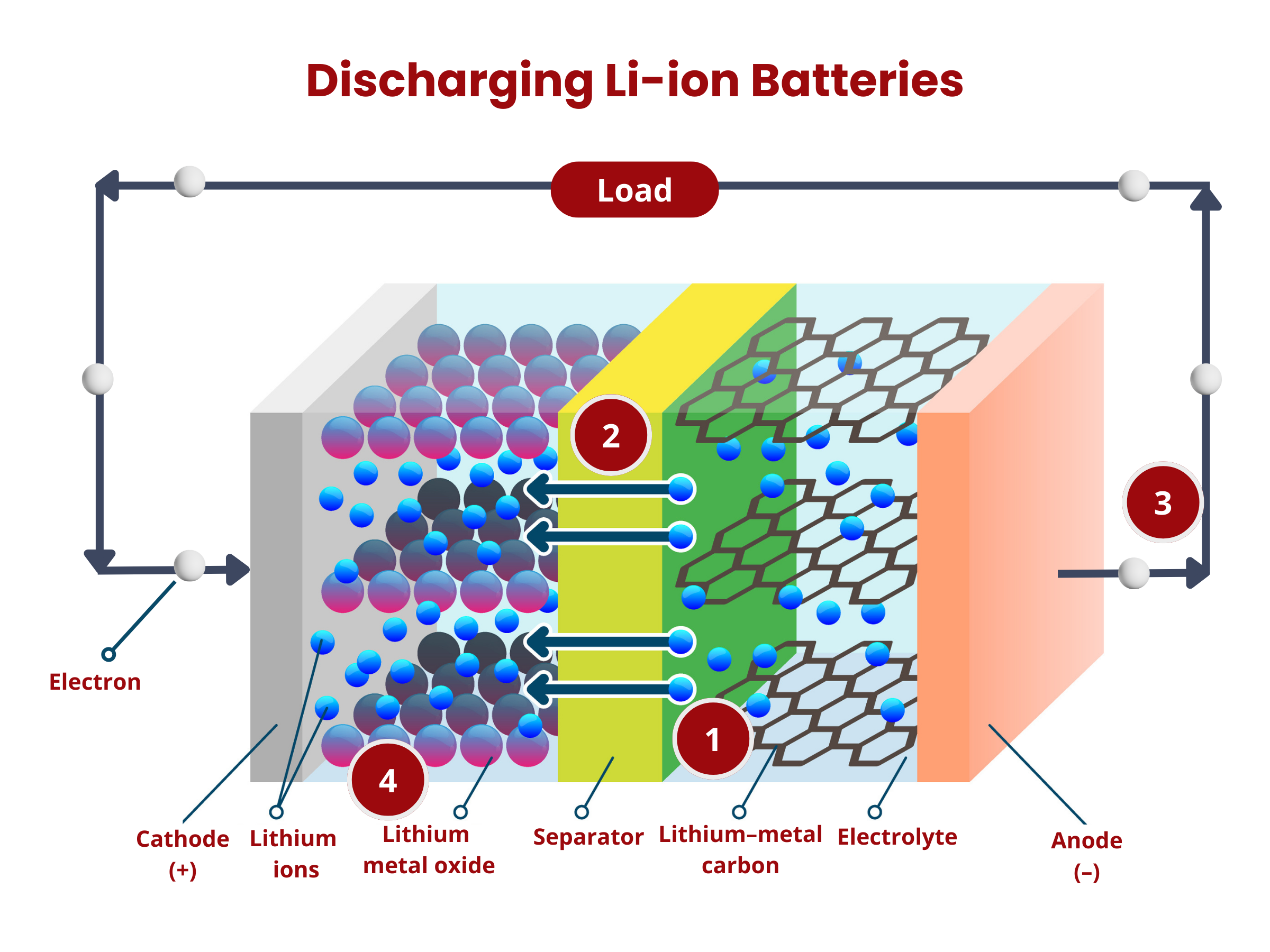
- Oxidation occurs at the anode. The graphite intercalation compound LiC6 is broken down into graphite (C6) and lithium ions, according to the reaction:
LiC6 → C6 + Li+ + e- - The lithium ions move through the electrolyte to the separator until they reach the positive electrode.
- The electrons produced in (1) move from the anode to the cathode through the external circuitry (wiring).
- Reduction occurs at the cathode using the electrons and lithium ions produced in (1). Cobalt oxide (CoO2) reacts with the lithium ions, producing LiCoO2 according to the reaction
CoO2 + Li+ + e- → LiCoO2
- The applied voltage forces the electrons to move from the cathode to the anode. This reverses the reduction reaction during the discharge process, causing the deintercalation of lithium ions from the cathode material:
LiCoO2 → CoO2 + Li+ + e- - The electrons move from the cathode to the anode through the external circuitry (wiring).
- The lithium ions move through the electrolyte to the separator until they reach the negative electrode.
- Intercalation happens at the anode, where lithium ions are inserted back into the anode material (graphite C6), according to the reaction:
C6 + Li+ + e- → LiC6
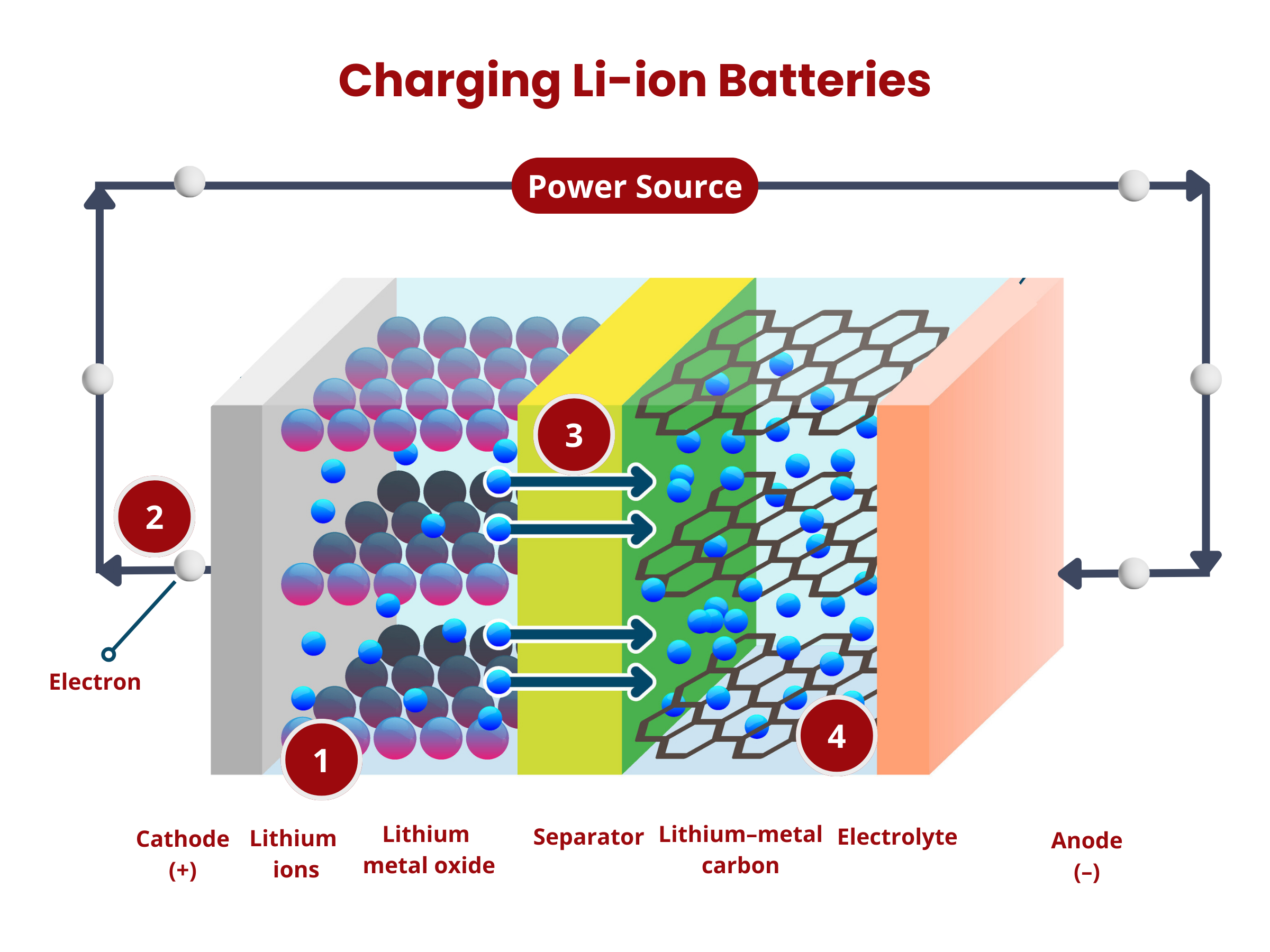
What are the battery cell components?
An electrochemical cell, or simply cell, is the basic electrochemical unit of batteries. In these cells, the conversion of chemical to electrical energy through the reduction and oxidation of electrochemically active materials occurs.
Cells are composed of these four major components: (1) positive and (2) negative electrodes, (3) electrolyte, and (4) separator.2
Battery cells have positive (cathode) and negative (anode) electrodes. During the discharging of secondary battery cells, electrons from an external circuit go to the cathode, where they drive the reduction of the electrochemically active material. On the anode, oxidation occurs, producing the needed electrons for the reduction reaction on the cathode. Both electrodes are composed of two primary components: an active material and a substrate or current collector.
The electrolyte in a battery facilitates the movement of charged ions between the cathode and anode, enabling the charging and discharging processes. It usually comes in liquid or paste form. The electrolyte's primary function remains consistent across various battery types. It serves as the medium for transporting charged ions, a crucial function in the overall operation of the battery.
In battery design, a separator, acting as an electrical insulator, prevents direct contact between positive and negative electrodes while facilitating the transfer of ions (ionic conductivity). The electrodes, separator, and electrolyte are assembled within a case or container, with terminals providing electrical connections to the external circuit. For safety, cells may include features like valves or current interrupt devices. Manufacturers have introduced various separator materials, with notable progress occurring in the second half of the 20th century in the chemical industry. Battery separators are categorized based on physical and chemical characteristics, composition, and structure, resulting in types such as nonwoven, microporous, ion-exchange, and nanoporous separators.
References
1 Mohammad Ali Abdelkareem, Mohamad Ayoub, Siren Khuri, Abdul Hai Alami, Enas Taha Sayed, T D Deepa, A.G. Olabi, Environmental aspects of batteries, Sustainable Horizons, Volume 8, 2023, 100074, ISSN 2772-7378.
2 Energy storage for electricity generation. (2023, August 28). U.S. Energy Information Administration (EIA). https://www.eia.gov/energyexplained/electricity/energy-storage-for-electricity-generation.php
3 Xu, Yan Wang, Yu Zhang, Cuo Li, Zhengmao. (2022). Coordination of Distributed Energy Resources in Microgrids - Optimisation, Control, and Hardware-in-the-Loop Validation - 1.2.2.1 Electrochemical Energy Storage. Institution of Engineering and Technology (The IET).
4 Ter-Gazarian, Andrei G.. (2020). Energy Storage for Power Systems (3rd Edition) - 9.1 General Considerations. Institution of Engineering and Technology (The IET).
5 Olsson, Gustaf. (2018). Clean Water Using Solar and Wind - Outside the Power Grid - 10.3.4 Flow Batteries. IWA Publishing.
Frequently Asked Questions about Batteries
The battery market is categorically divided into consumer, automotive, industrial, and special applications, which include aerospace and military sectors.
In consumer electronics, lithium-ion batteries have become the major rechargeable power sources due to their high energy density, lightweight nature, and long cycle life. This chemistry is favored for its ability to deliver sustained and reliable performance in devices such as smartphones, laptops, cameras, and portable electronic gadgets. Automotive applications traditionally use batteries for Starting, Lighting, and Ignition (SLI), with lead–acid being the exclusive chemistry employed. Newer automotive systems, such as Hybrid Electric Vehicles (HEV), Plug-in Hybrid Electric Vehicles (PHEV), and Electric Vehicles (EV), employ other battery chemistries. The industrial sector further classifies batteries for mobile and stationary applications. This segmentation underscores the varied and evolving requirements across sectors within the battery industry.
A battery is composed of one or more of these electrochemical cells that are electrically connected to achieve the required operating voltage or current. Therefore, the term “battery” can be used to denote a single-cell or multi-cell battery. In addition to cell components, batteries have various elements, such as control and monitoring components, case/container, cell connectors, markings, and supplementary equipment like fuses, communication systems, as well as cooling and venting systems.
Electric vehicles (EVs) often require more than one battery (or battery module) to achieve the necessary operating voltage and current levels. In such cases, EV battery packs are used. Battery packs are the larger units that house and interconnect multiple modules, providing the overall energy storage for the vehicle, whereas a battery module is a self-contained unit consisting of several individual battery cells. The hierarchy is often as follows: individual cells → modules (batteries) → packs. Learn more information about materials for EV battery packs here.