Ion Exchange Membranes & Polymers
Ion exchange membranes and polymers enable the development of next-generation environmental technologies. These materials have a hydrocarbon backbone, which enables performance and life cycle improvements across the industry in fuel cells and hydrogen electrolysis, while making them far less impactful on the environment than common fluorinated materials. By using advanced stabilization techniques, they are able to compete with the most robust of alternatives.
Hydrocarbon membranes are significantly tougher than competitive products leading to a longer service life and reduced lifetime cost. Unlike their proton exchange counterparts, alkaline based anion exchange membrane systems can operate without the use of precious metals such as platinum and iridium, making them way more cost effective and environmentally friendly. Caplinq is proud to present the ONLY High performance non-fluorinated material in the market.
PEMION - PF1-HLF8-15
- PFAS-free Proton Exchange Membrane with Hydrocarbon reinforcement
- 15 µm thickness
- Optimized for heavy duty fuel cell applications
- 4 - 6 weeks
AEMION+™-AF3-HWC9-70
- Water Electrolysis
- Acid Recovery and Salt Splitting
- 70 micron thick membrane with woven PEEK reinforcement
- 4 - 6 weeks
AEMION+™ - AF3-HWK9-75
- Water Electrolysis
- Acid Recovery and Salt Splitting
- 75 micron thick membrane with woven PEEK reinforcement
- 4 - 6 weeks
PEMION+™ - PP1-HNN8-00
- High conductivity binder and coating material
- High concentration of functional groups
- Chemical and oxidative stability
- 4 - 6 weeks
AEMION+™ - AP3-HNN9-00
- Enabling precious metal free systems
- High conductivity
- Catalyst layer ionomer
- 4 - 6 weeks
Product Selector Guide
Product | Type | Thickness (um) | Tensile strength (MPa) | Young's Modulus (MPa) | Elongation (%) | Water uptake (%) | Max process temp (°C) | Reinforced |
---|---|---|---|---|---|---|---|---|
AF3- CLF9-25 | Anion | 25 | > 85 | > 480 | > 80 | <15 | 150 | NW PTFE |
AF3- CLF9-50 | Anion | 50 | > 80 | > 450 | 80 - 120 | <15 | 150 | NW PTFE |
AF3-HWK9-75 | Anion | 75 | > 57 | > 630 | 27 | <15 | 150 | Woven PEEK |
PF1- HLF8-15 | Proton | 15 | > 50 | > 600 | > 50 | 150 - 190 | 160 | Hydrocarbon |
Product | Type | IEC (meq/g) | Conductivity (mS/cm) | Water uptake OH- % | Density (g/cm3) | Solubility |
---|---|---|---|---|---|---|
AP3-HNN9-00 | Anion | 1.9 - 2.7 | 4 - 9 | 20 - 50 | - | - |
PP1-HNN8-00 | Proton | 2.8 - 3.1 | - | - | 1.2 | Alcohols/Water |
- IEC in the hydroxide (OH-) counter-ion form.
- Approximate swelling properties when cast into membrane form at 25 - 50 μm
These are prototype materials only intended to be used for early development activities and not intended for production items. Product information is to be used as a guide only, subject to change at any time.
Frequently Asked Questions
What is an ION Exchange material?
Ion Exchange Polymers (Ionomers)
ION Exchange polymers are at the core of all electrochemical technologies. They are being used as a coating/binder in the manufacturing of electrodes that are required for electrochemical systems
They allow rapid access to reactive sites, improving the application\'s efficiency, rate and capacity. Anion exchange polymers in particular combine the benefits of liquid alkaline and PEM systems while improving on almost every single aspect when it comes to Electrolysis. They have a very low H2 crossover, they don\'t require precious metals or titanium and they have a very high volumetric efficiency.
Ion Exchange Membranes
ION Exchange membranes are being used as a chemical barrier for efficient energy conversion systems.
These materials selectively filter positive or negative ions, including acid and base. The most popular membranes right now are the Proton exchange membranes but the market is shifting towards cheaper, more efficient and enviromentally friendly solutions. Anion exchange membranes are the next best thing due to their cost efficiency and they are bound to completely shake the energy market in the following years.
Learn More
What are the Key Properties of Ion Exchange Membranes?
Generally, the properties of IEMs are determined by the properties of the polymer backbone and fixed charges that make up their structures. Particularly, the density, wettability (hydrophobicity or hydrophilicity), and morphology of the polymer matrix, as well as the type and concentration of the charged functional groups, affect the resulting properties of the IEMs. The mechanical, chemical, and thermal properties of IEMs are primarily influenced by the polymer backbone, whereas the electrochemical properties, conductivity, and permselectivity are determined by the concentration of the fixed charges.
Ion Exchange Capacity. IEC is the concentration of the fixed charged species measured as milliequivalent per gram (meq (fixed charge)/g (dry IEM)). The IEC of most commercially available IEMs varies from 1–3 meq/g. IEC is an important material property because it affects other IEM properties, such as ionic conductivity, swelling ratio, water uptake, and fluid permeability.
Ionic conductivity. Ionic conductivity is a quantitative measure of the electric-field induced transport of ions across the IEM. It is the inverse of the membrane specific resistivity, which is an intrinsic IEM property that can be used for the comparison of the inherent efficiency of different IEMs. To put it simply, ionic conductivity tells how well the membrane can facilitate the transport of the counter-ions across. It is related to the IEC of the membrane. Higher IEC means that there are more functional groups in the polymer backbone that can participate in the ion transport, which potentially increases the ionic conductivity of the IEM. In turn, this higher ionic conductivity reduces the overpotentials and improves the performance and efficiency of the water electrolysis and fuel cells. While it is affected by the IEC, ionic conductivity is also influenced by other factors, such as membrane morphology, water content, and membrane thickness.
Permselectivity. Permselectivity is one of the most important properties of IEMs. It refers to the ability of the membrane to selectively allow the transport of the counter-ions while blocking the passage of the co-ions. Permselectivity values range from 0 to 1. For example, a perfectly selective PEM (with a permselectivity of 1) will only allow the diffusion of protons (H+ ions) across the membrane, completely disabling the transport of negatively charged species. On the other hand, permselectivity <1 implies that the IEM allows the passage of some co-ions. Highly permselective IEMs ensures that (1) the required ions for the electrochemical reactions are transported with greater efficiency, (2) the crossing over of species at the anode and cathode is minimized. The crossing over of reactants and products between the electrodes decreases the overall efficiency of the electrochemical device as less reactant is supplied at the site at which it is needed. Another thing, the crossover of ions can yield chemical reactions that cause corrosion or degradation of the IEM and other components. Highly permselective membranes are desired to achieve high performance, maintain product purity, ensure operational safety, and extend the longevity of the electrochemical device.
Dimensional stability (Membrane swelling and water uptake). IEMs are used to transport counter-ions while disabling the diffusion of co-ions and other redox-active species in electrochemical energy storage technologies. At the same time, IEMs must regulate the water (or other solvent) transport, keeping in mind that IEMs need to be hydrated to function properly. Dehydration can lead to the loss of ion conductivity, membrane shrinkage, and a decrease in overall performance. Proper hydration helps prevent these issues and ensures the long-term stability of the membrane. But, a striking balance between membrane hydration and water removal must be achieved. Too much water uptake (or water content) in the IEM might lead to membrane swelling, which affects the dimensional stability of the IEM. Flooding can ensue in which the excess water can block the transport pathways.
Membrane swelling measures the degree at which the membrane expands or its volume increases as the IEM absorbs water. Water uptake, on the other hand, measures the amount of water that an IEM can absorb under specific conditions. Optimal levels of membrane swelling and water uptake is required for effective water management in the device.
Chemical, mechanical, and thermal stability. Aside from performance-related properties, the chemical, mechanical, and thermal stability of IEMs are equally important to ensure the durability and long-term performance of fuel cells and water electrolyzers. Chemical stability is important to prevent membrane degradation as they are subjected to very aggressive conditions during operation. Mechanical stability ensures that the membrane will not fail in response to the compressive force applied to produce the stack. Lastly, thermal stability ensures that the IEM can continue to perform and not fail due to temperature effects.
What are Anion Exchange membranes?
Anion Exchange Membranes (AEMs) are a specialized type of ion exchange membrane that are specifically designed to exchange anions (negatively charged ions) in electrochemical processes. They are used in various applications such as water treatment, fuel cells, and batteries, where it is important to regulate the flow of anions. Some of the key features and benefits of AEMs include:
High selectivity: AEMs are designed to selectively exchange anions, ensuring the stability and efficiency of electrochemical reactions.
Improved performance: The use of AEMs can result in improved performance in electrochemical processes, particularly in fuel cells and batteries.
Customizable properties: The properties and characteristics of AEMs can be customized to meet the specific needs of different applications.
Durable and long-lasting: AEMs are designed to be strong and long-lasting, making them ideal for demanding applications.
AEMs are made of a polymer material that contains functional groups with a negative charge, such as sulfonic acid or carboxylic acid. These functional groups are capable of exchanging anions in electrochemical processes. The structure of the membrane and the type of functional group can affect the properties and characteristics of the AEM, including its ion exchange capacity, selectivity, and stability.
In electrochemical processes, AEMs are typically used to separate anions and cations in a flow field, allowing for the regulation of ionic flow. This regulation can result in improved performance and efficiency, particularly in applications such as fuel cells and batteries, where the exchange of anions is critical.
AEMs can also be customized to meet the specific needs of different applications. For example, the type and concentration of functional groups can be adjusted to improve the selectivity of the membrane, and the thickness and structure of the membrane can be optimized to improve its stability and durability.
Durable Anion Exchange Membranes
Aemion™ anion exchange membranes are the most durable and high-performance anion exchange products on the market. They are stable in both highly basic and strongly acidic environments, providing a marked improvement in product life cycle, even in the harshest operating conditions. This unique stability unlocks a broad range of applications, from fuel cells, chemical generation, recovery and long-life energy storage to electrolysis and hydrogen production.
These membranes are based on the breakthrough discovery that weak sites in polymer structures are the root cause of AEM instability, and they can be effectively protected to prevent alkaline and oxidizing species from breaking down the polymers. The resulting hydrocarbon backbone provides a platform that enables simultaneous performance and life cycle improvements across the industry in energy and water, while reducing your product’s environmental impact.
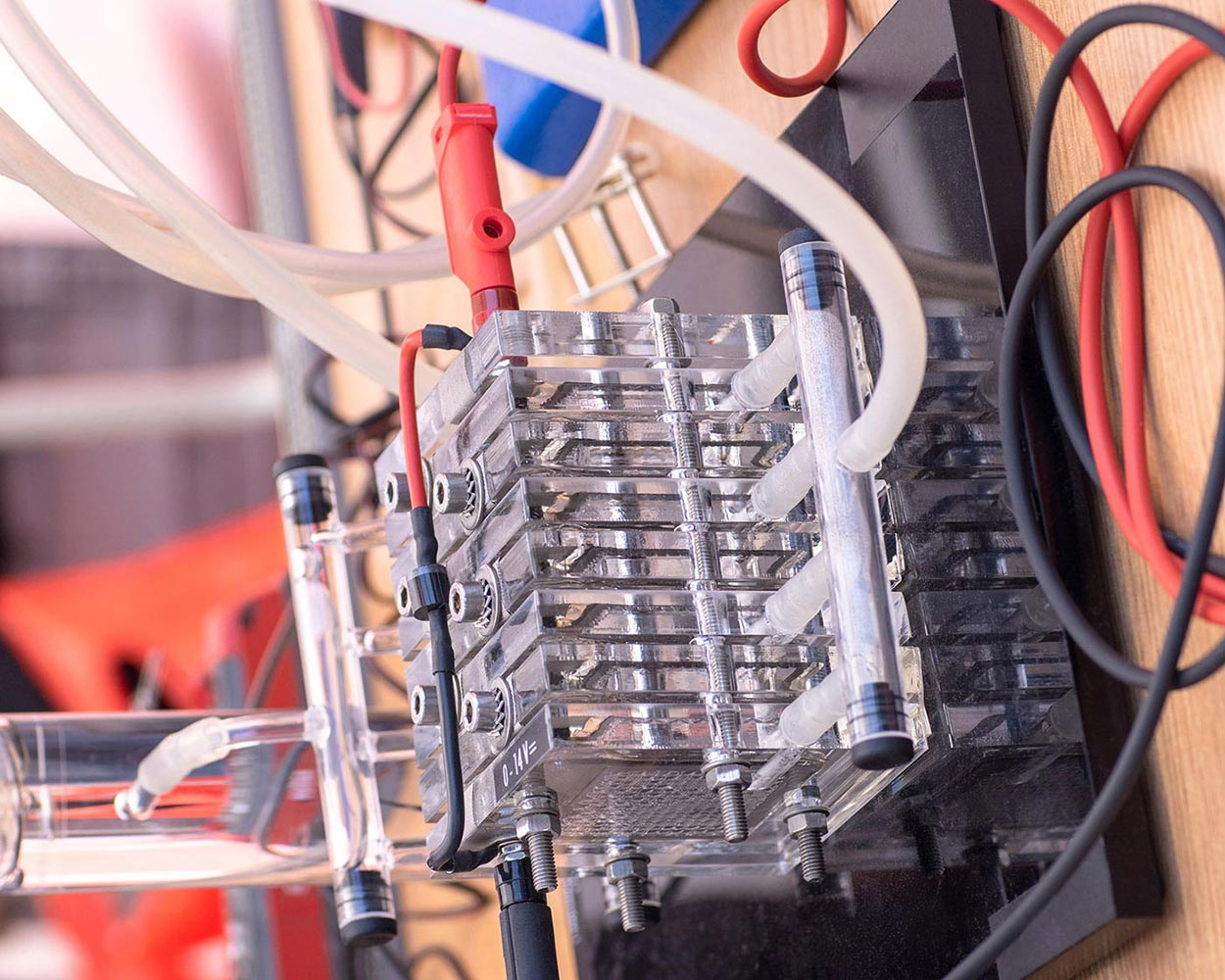
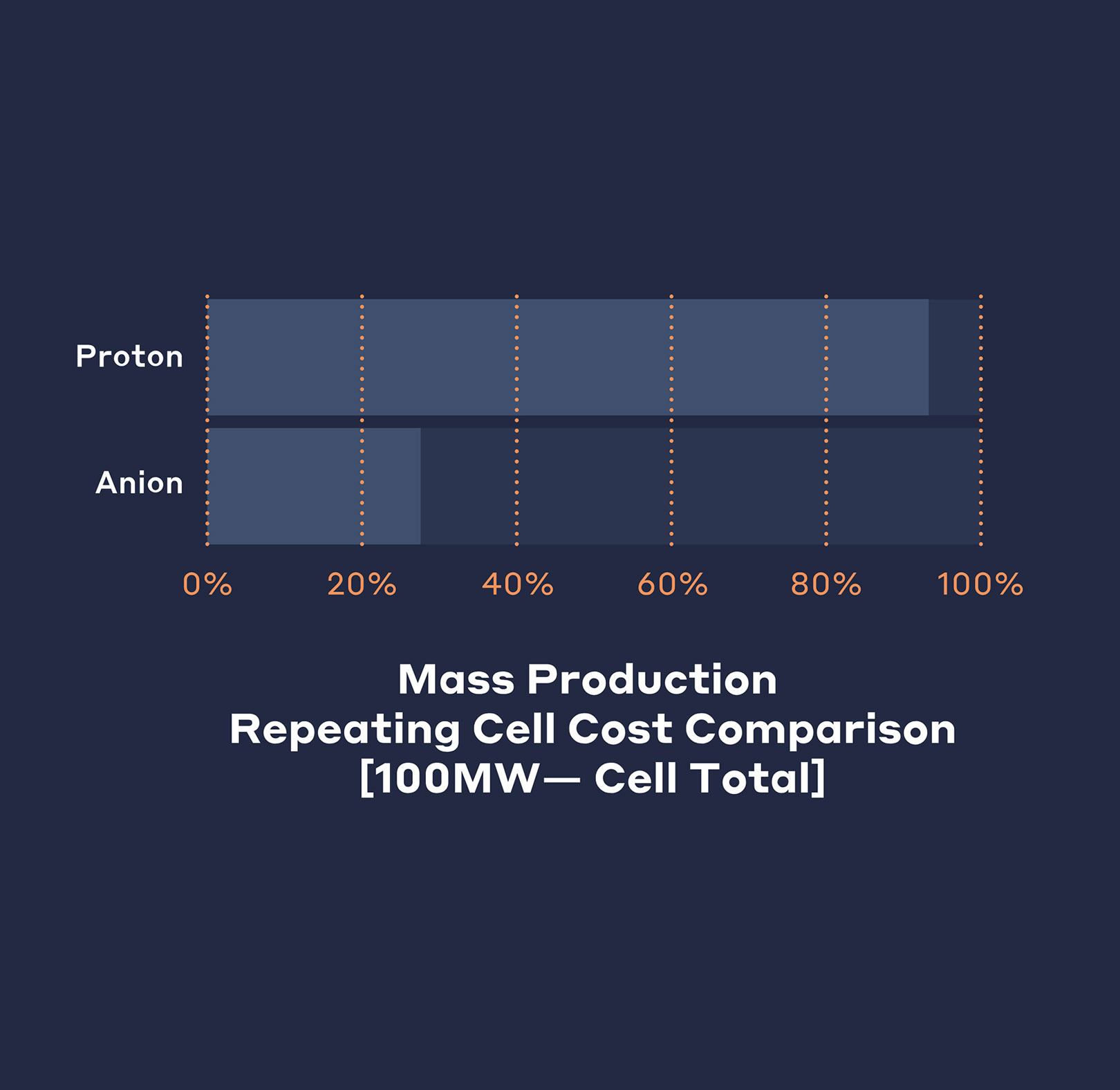
Stable
Chemically and oxidatively stable across a range of operating conditions. A product that provides strong alkaline functionality (>2M @ 60ºc) in addition to acidic stability for use in the harshest of applications.
High Strength
Strongest unsupported materials on the market for ease of handling, longer life and thinner membranes, resulting in low ionic resistance.
Performance
High conductivity at both room and elevated temperatures to enable low resistance applications with higher current densities.
Processable
Soluble polymer in low-boiling solvents, allowing simple and consistent integration into existing product platforms as a strong industrial coating. Can be used as a stable, ion-conductive catalyst binder and enables coated, 3D electrodes
Green
Reduces the environmental impact of your product throughout its entire lifecycle.
Anion Exchange Membrane applications
Hydrogen Electrolysis
Next gen anion exchange membranes were developed specifically for use in hot and strong alkaline environments and are the leading solution available for the evolution of low cost, high efficiency alkaline membrane electrolysis systems. The high hydroxide ion conductivity enables high efficiency and energy density, which lead to overall opex and capex reductions, while the chemical and mechanical integrity leads to longer operational usefulness.
An AEM electrolysis solution combines the benefits of PEM and alkaline systems by allowing the use of non-noble catalysts while achieving energy densities and efficiencies comparable to PEM technology. Today, commercially available membranes lack sufficient stability in alkaline, which has limited and the widespread adoption of AEM in electrolysis applications.
The cost of precious metals such as platinum and iridium dwarf those of commodity metals such as nickel and iron. AEMs will unlock the potential for massive cost savings and scalability currently limited by precious metal supply. The charts highlight just how extreme this difference is and the reductions in catalyst cost possible.
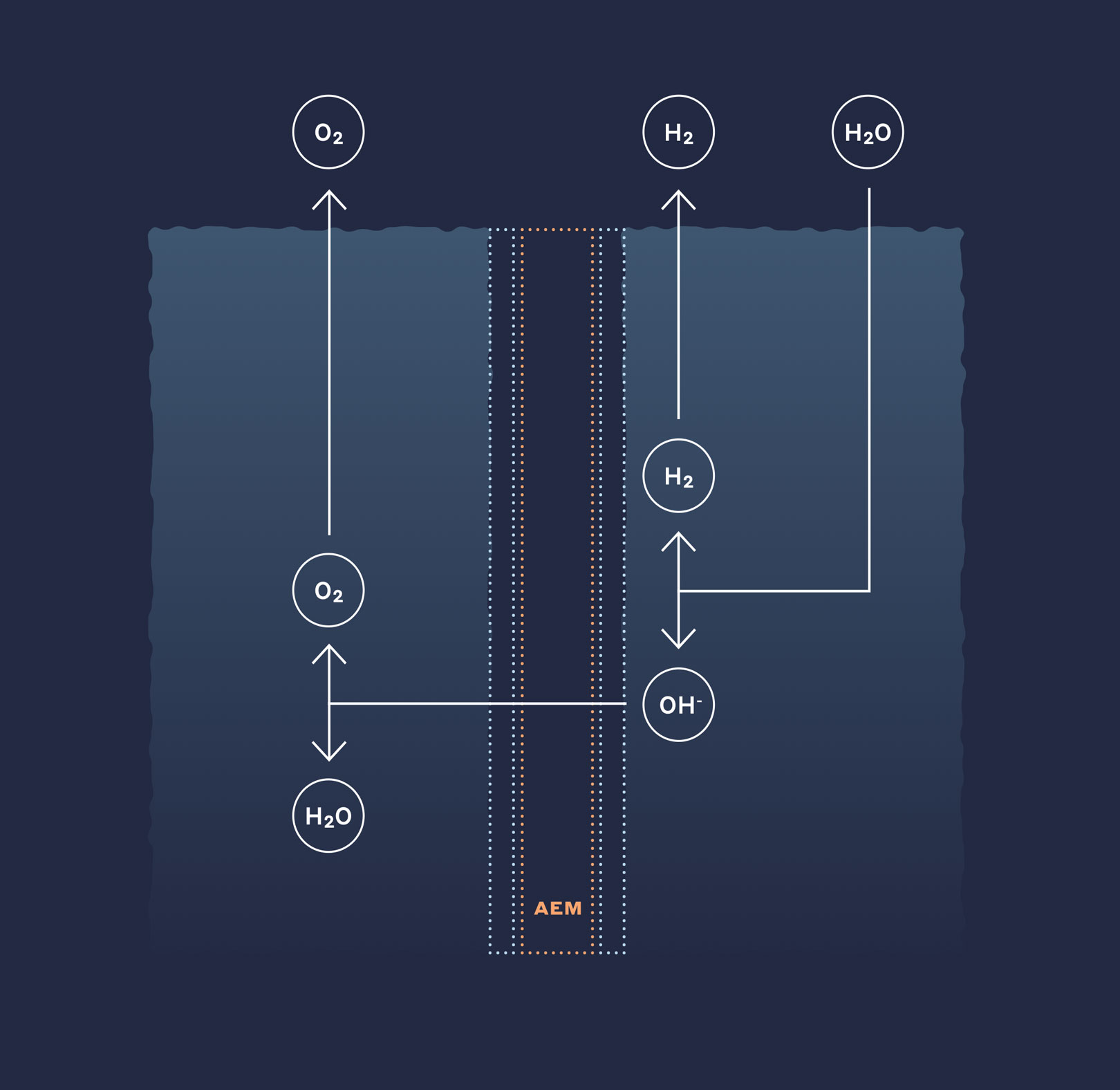
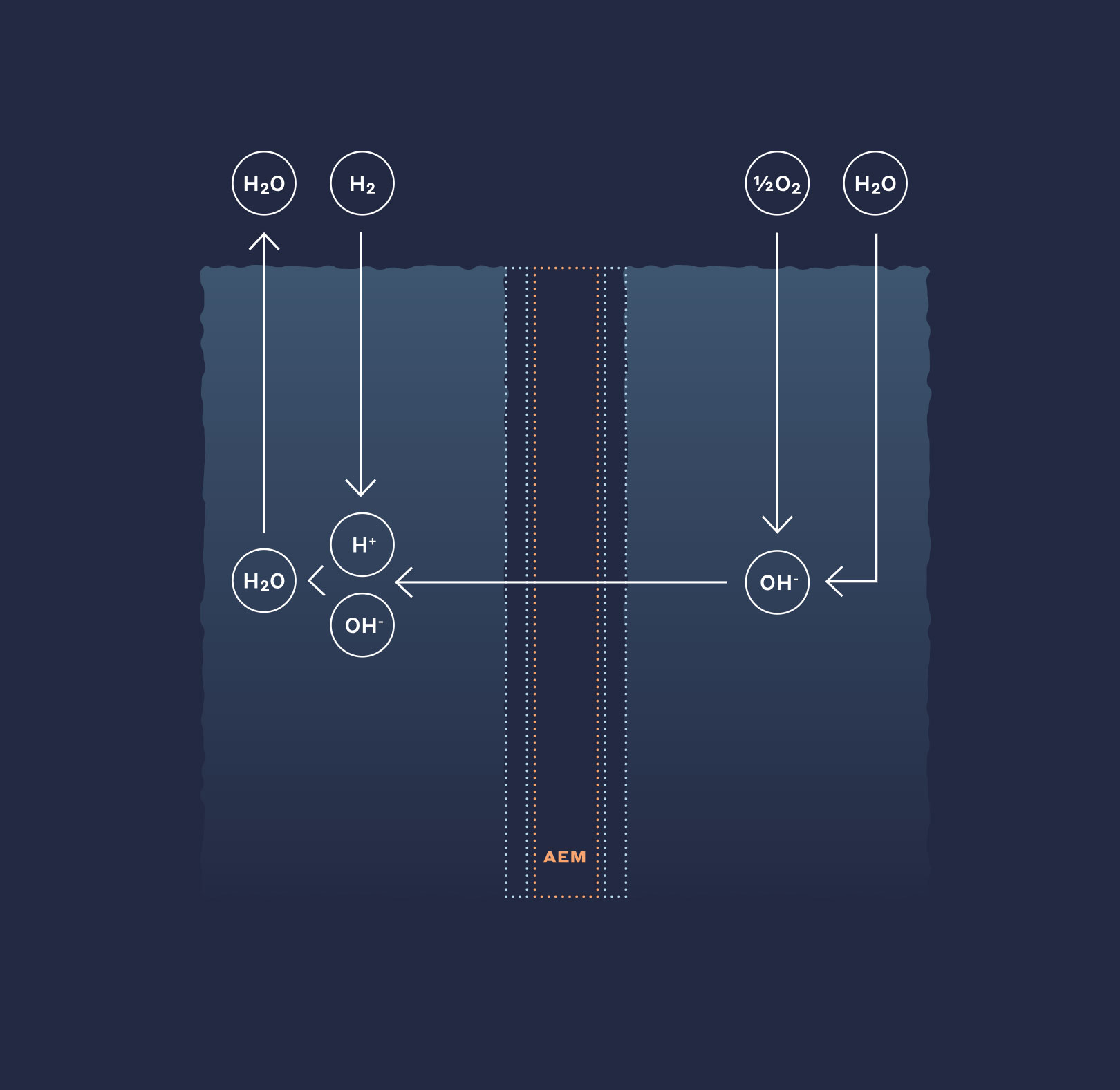
Hydrogen Fuel Cells
Anion exchange membranes were developed for stability in strong alkaline solutions under the oxidizing conditions of high voltage and current. They represent one of the leading solutions available for continued development in next-generation, low cost, high efficiency fuel cells.
Current generation fuel cells require expensive precious metal catalysts such as platinum to operate effectively since commodity metals simply cannot function in, or withstand the harsh acidic environment of the fuel cell.
Anion exchange membranes enable OEM fuel cell developers to scale up lower cost innovative system architectures that do not require precious metals. Using alternative liquid fuels, AEM fuel cells significantly increase the power density over existing PEM-based systems. Capable of decreasing the system cost by as much as 80%, platinum-free fuel cells are cost-competitive with combustion engines and achieve the minimum environmental impact for any power system.
Anion exchange membranes are making Fuel cells a sustainable solution once again and we are looking forward to help you test and experiment with this upcoming technology that can potentially change the future of energy as we know it.
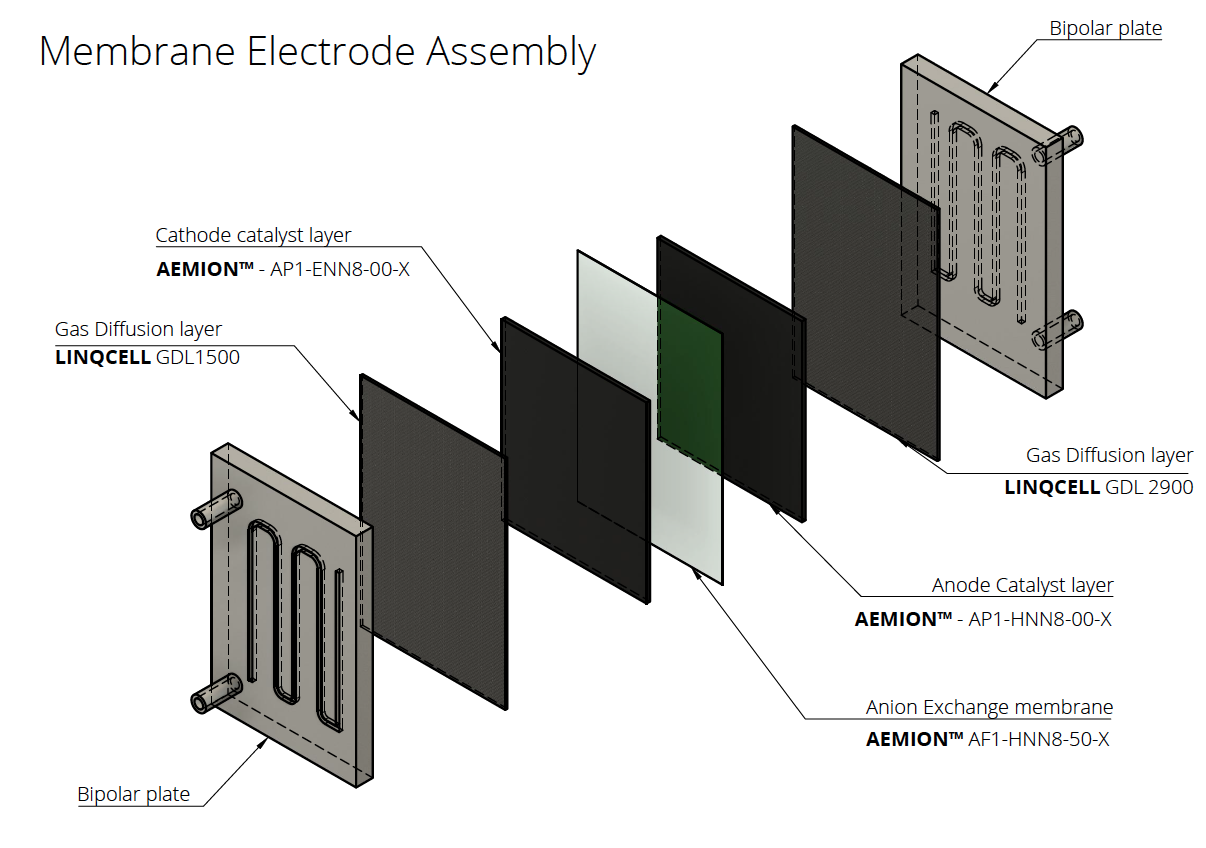
What are Ionomer powders?
Ionomer powders are a type of thermoplastic material that are widely used in several applications across various industries. These materials are characterized by their ability to conduct ions and offer a combination of mechanical and electrical properties, making them ideal for use in a variety of applications, such as:
Fuel Cells: Ionomer powders are used as a key component in the membrane electrode assembly (MEA) of fuel cells. The ionomer acts as a binder to hold the catalyst particles in place and also provides an ion-conducting path between the electrodes.
Battery Separators: Ionomer powders are used as separators in rechargeable batteries to prevent the electrodes from coming into direct contact, which would cause a short circuit. The ionomer's electrical conductivity and ability to conduct ions make it an ideal material for this application.
Coatings: Ionomer powders are used as a binder in coatings to improve adhesion, toughness, and abrasion resistance. These materials are commonly used in the coating of golf balls, where they provide an ideal balance between durability and softness.
Medical Devices: Ionomer powders are used in medical devices such as implants and sutures to improve strength, toughness, and biocompatibility. The materials are also used as coatings on medical devices to improve their biocompatibility and reduce the risk of adverse reactions.
Packaging Films: Ionomer powders are used in packaging films to improve strength, toughness, and heat resistance. These materials are commonly used in the production of shrink wrap and stretch wrap, where they provide an ideal balance between flexibility and durability.
In conclusion, ionomer powders have a wide range of applications, making them an essential component in several industries. Their combination of mechanical, electrical, and ion-conducting properties make them ideal for use in fuel cells, battery separators, coatings, medical devices, and packaging films.